小型デジタルサーボコントローラー
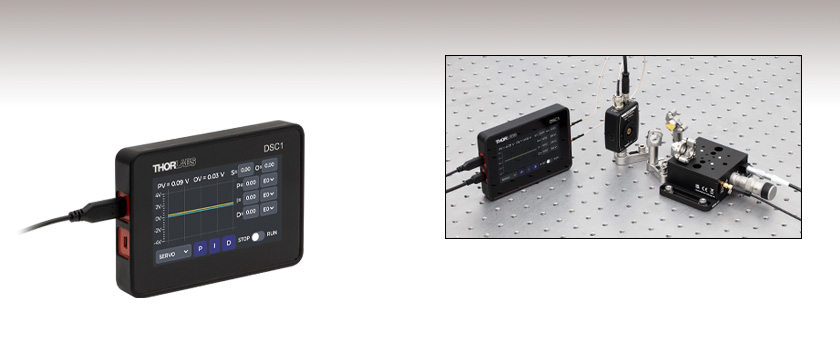
- Digital Servo Controller with 100 kHz Bandwidth
- Servo Lock, Peak Lock, and Ramp Function Modes
- Ideal for Frequency Stabilizing Lasers
- Compact Housing with a Touchscreen Interface
Application Idea
A DSC1 Compact Digital Servo Controller can be used to lock an interferometer to the peak or side of a single interference fringe by taking an input from a photodiode measuring the interferogram. The output of the DSC1 controller can then be passed to a piezo driver controlling a dynamic arm of the interferometer.
DSC1
Compact Digital Servo Controller
(Power Supply Sold Separately)

Please Wait
Key Specificationsa | |
---|---|
System Bandwidth | DC to 100 kHz |
Input-to-Output -180 Degree Frequencyb | >58 kHz (60 kHz Typical) |
Nominal Input Sampling Resolution | 16 Bit |
Average Noise Floor | -120 dB V2/Hz |
Peak Noise Floor | -105 dB V2/Hz |
Input RMS Noisec | 0.3 mV |
Maximum Input Voltage | ±4 V |
Maximum Output Voltaged | ±4 V |
Dimensions (L x W x H) | 129.8 mm x 91.3 mm x 21.6 mm (5.11" x 3.59" x 0.85") |
用途例
- 単一周波数レーザまたは共振器のロック
- 干渉計の安定化
- フーリエ変換分光法
- 飽和吸光分光法
- ダイクロイック原子蒸気レーザーロック(DAVLL)
- アクティブビームポインティングの安定化
- レーザーパワーの安定化
- レーザの繰返し周波数ロック
- 外部共振器半導体レーザ(ECDL)
- ファイバ位相または光路長の安定化
- CW SHG共振器用のエンハンスメント共振器ロック
- AOMによる強度安定化(ノイズ除去)
- モーションコントロール
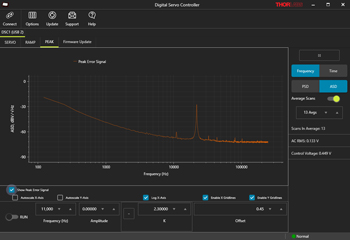
Click to Enlarge
デジタルサーボコントローラ用ソフトウェアは、コントローラDSC1用のシンプルで使いやすいインターフェイスです。入力電圧は周波数領域または時間領域で表示できます。
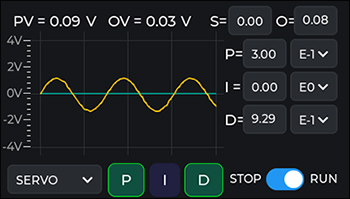
特長
- 最大帯域幅100 kHzの高速PID制御
- 周波数安定化レーザに対応したレーザーロックボックス
- USB Type-Cで駆動する幅130 mmの小型筐体
- タッチパネルからすべての機能にアクセス可能(詳細は「タッチスクリーン」 タブ参照)
- 3つのモード
- Servo Lock:規定の入力電圧にロック
- Peak Lock:ディザロック(ピークロッキング、Extremum Seeking制御、ロックイン安定化とも呼ばれます。)
- Ramp:出力電圧を設定範囲にわたりリニアに掃引
- デジタルサーボコントローラ用ソフトウェアを使用して信号の追加解析が可能(「ソフトウェア」タブからダウンロードいただけます)
- Servo Lockモード:入力電圧を時間領域または周波数領域で表示
- Peak Lockモード: 復調された入力電圧を時間領域または周波数領域で表示
- Rampモード:入力電圧をオシロスコープのようにランプ出力電圧の関数として表示
- デジタルサーボコントローラのフルコントロールに使用可能
当社の小型デジタルサーボコントローラDSC1は、ユーザが選択した制御アルゴリズムによって決定されるリアルタイム電圧フィードバックを提供します。そのフィードバックにより、入力電圧信号に対して光学システムをロックまたは安定化するような設計となっています。帯域幅は100kHz、入出力電圧範囲は±4Vで、電流変調レーザ、ピエゾアクチュエータ、音響光学変調器(AOM)、電気光学変調器(EOM)、ファイバーストレッチャ、ボイスコイル、高速ステアリングミラーなどの高周波デバイスをアクティブにロックすることができます。
このコントローラには、補完的な機能を備えた3つのモード(Servo Lockモード、Peak Lockモード、Rampモード)があります。
Servo Lockモードを使用する場合、出力電圧をPID(比例、積分、微分)アルゴリズムで変調し、設定された入力電圧を維持することができます。アルゴリズムのすべてのパラメータは、特定のシステム用に調整することができます。例えば、フォトダイオードでパワーを測定し、フォトダイオードからの信号をサーボコントローラに入力し、サーボコントローラからの出力で音響光学変調器(AOM)、電気光学変調器、LN変調器などの強度変調器を制御することで、システムの出力強度を安定化させることができます。サーボコントローラのセットポイントを変更すると、システムはその新しいセットポイントに調整され、維持されます。 さらに、サーボコントローラのServo Lockモードを使用して、サイドフリンジロックでレーザの周波数を安定させることができます。サイドフリンジロックでは、ファブリペロー共振器からの共振ピーク、または原子や分子からの吸収ピークの途中にセットポイントを置くことができます。
Peak Lockモードでは、ディザロック(Extremum Seeking制御またはロックイン復調サーボとしても知られています)を使用して、入力電圧の局所的な最大値または最小値にシステムをロックします。これは、選択した周波数(ディザ周波数)でシステムを変調し、その信号をデジタル復調して、最小化できる誤差関数を作成することによって行われます。入力電圧の最大値または最小値にシステムをロックすることにより、システムの性能を厳密に制御することができます。例えば、レーザの周波数をアセチレンのような希薄ガス中の分子吸収線にロックしたり、レーザーパワーのドリフトや変動に対して固有の耐性を持つファブリペロー共振器の最大透過モードにロックすることができます。詳細については「レーザのロック」タブをご覧ください。
Rampモードでは、デジタルサーボコントローラは、設定した範囲にわたって出力電圧をリニアに掃引し、その結果得られた入力信号をオシロスコープのように電圧の関数として表示することができます。これにより、干渉計からのインターフェログラムのような特徴を解析できるだけでなく、印加電圧範囲におけるシステムの応答を直接確認することができます。例えば、サーボコントローラがガス吸収セルを通過するレーザの周波数を掃引すると、ガスの透過スペクトルを観察することができます。
使用例
デジタルサーボコントローラによって制御されるピエゾ駆動のミラーを備えた干渉計をロックするような用途では、インターフェログラムを測定するフォトダイオードからの信号がサーボコントローラに入力されます。サーボコントローラをServo Lockモードに設定すると、干渉計は干渉縞の1本のフリンジにおける正または負の傾きの任意の閾値にロックされます。サーボコントローラをPeak Lockモードに設定すると、干渉計はインターフェログラム内の1本の干渉縞のピークまたはバレーにロックされます。サーボコントローラをRampモードに設定すると、インターフェログラムを直接観察することができます。
タッチパネル
小型デジタルサーボコントローラDSC1のタッチパネル式インターフェイスは、3つのモードすべてでフル機能をご使用いただけます。これには、制御アルゴリズムの実行と停止、パラメータの変更、時間の関数としての入力電圧のグラフの表示が含まれます。詳細については、「タッチパネル」タブをご覧ください。
ソフトウェア
デジタルサーボコントローラDSC1は、デバイスの左側にあるUSB Type Mini-Bポートを使用してPCに接続し、ソフトウェアを用いて制御できます。ユーザーフレンドリーなインターフェイスで、制御スキームの開始と停止、制御パラメータの変更、ロックパフォーマンスをモニタするための制御を行うことができます。時間領域と周波数領域の両方でグラフ表示できます。CSLソフトウェアの詳細については「ソフトウェア」タブをご覧ください。
電源
デジタルサーボコントローラDSC1は、デバイス左側にあるUSB Type-Cポートから電源供給できます(「ピン配列 tab)」タブ参照)。USB Type-A - USB Type-Cの電源ケーブルが付属していますが(「発送品リスト」タブ参照)、電源は別売りです。
接地
低周波数での測定出力時に不規則な動作を引き起こす可能性がある接地ループを避けるようご注意ください。接地ループの経路が形成される可能性を避けるため、USB Type-Cの電源ポートはデバイスの接地面に接続して、USB Type-Mini-Bのデータポートはコントローラをコンピュータの接地面から絶縁するために浮かせます。コントローラDSC1を実験セットアップに統合する際の接地ループのリスクを軽減するための最良の方法については、マニュアルをご覧ください。
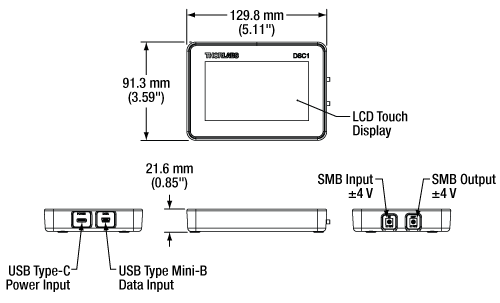
Click to Enlarge
小型デジタルサーボコントローラDSC1の寸法
Item # | DSC1 |
---|---|
Operating Specifications | |
System Bandwidth | DC to 100 kHz |
Input to Output -180 Degree Frequencya | >58 kHz (60 kHz Typical) |
Nominal Input Sampling Resolution | 16 Bit |
Nominal Output Resolution | 12 Bit |
Maximum Input Voltage | ±4 V |
Maximum Output Voltageb | ±4 V |
Maximum Input Current | 100 mA |
Average Noise Floor | -120 dB V2/Hz |
Peak Noise Floor | -105 dB V2/Hz |
Input RMS Noisec | 0.3 mV |
Input Sampling Frequency | 1 MHz |
PID Update Frequencyd | 500 kHz |
Peak Lock Modulation Frequency Range | 100 Hz - 100 kHz in 100 Hz Steps |
Input Termination | 1 MΩ |
Output Impedanceb | 220 Ω |
Dimensions | 129.8 mm x 91.3 mm x 21.6 mm (5.11" x 3.59" x 0.85") |
Electrical and Data Specifications | |
Supply Voltage from USB | 4.75 - 5.25 V DC |
Supply Current | 750 mA (Max) |
Temperature Rangee | 0 °C to 70 °C |
Touchscreen
The touchscreen interface on the DSC1 Compact Digital Servo Controller provides the ability to control all parameters in each of the three modes: Servo Lock, Peak Lock, and Ramp. The output can be started and stopped with the toggle in the bottom right corner of the screen. Additionally, the plot displays the input voltage as a function of time and, in Servo Lock mode, the set-point voltage.
List of Abbreviations on the Touchscreen:
- PV - Process Variable, Volts
- This is the voltage being fed into the input of the DSC1 controller via the input SMB terminal.
- OV - Output Variable, Volts
- This is the voltage being output from the DSC1 controller via the output SMB terminal.
- S - Setpoint, Volts
- O - DC Offset, Volts
- P - Proportional Coefficient
- I - Integral Coefficient, 1/Seconds
- D - Derivative Coefficient, Seconds
- M - Modulation Frequency Multiplier, x100 Hertz (e.g., For M=5, the Modulation Frequency is 500 Hz)
- A - Amplitude, Volts
- Peak Mode: Amplitude of the Sinusoidal Modulation Voltage
- Ramp Mode: Amplitude of the Linear Voltage Ramp, from -A to +A
- K - Peak Lock Integral Coefficient
Servo Lock Mode
When the Servo Lock mode is selected, the touchscreen can be used to set all of the parameters for the servo locking function. Pressing the P, I, or D button at the bottom of the screen enables and disables their contribution to the PID calculation. Pressing the numerical values on the right of the screen allows for the coefficient value to be changed. Changing the sign of the P, I, and D coefficients switches from locking on a positive slope to a negative slope and vice versa. The setpoint and DC offset can be changed by pressing the numerical values at the top of the screen. The golden line is a display of the input voltage and the teal line is the set point.
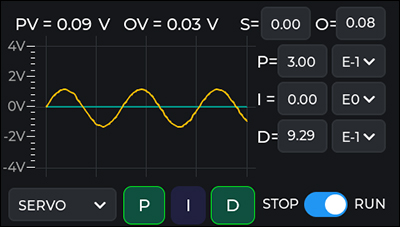
Peak Lock Mode
When the Peak Lock mode is selected, the touchscreen can be used to set all of the parameters for the peak locking function. Pressing the numerical values on the right of the screen allows for the coefficient values of M, O, A, and K to be changed. Changing the sign of K switches from locking on a peak to a valley and vice versa. The golden line is a display of the demodulated input voltage, the error signal in the locking algorithm.
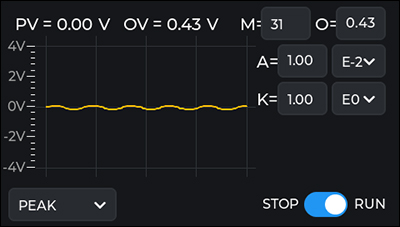
Ramp Mode
When the Ramp mode is selected, the touchscreen can be used to set all of the parameters for the ramp function. Pressing the values of O and A allows for the coefficient values to be changed. The golden line is a display of the input voltage as a function of the ramped output voltage, -3 V to 3 V in the example to the right. Note that the plot on the touchscreen interface is down-sampled and may not show fast features in the system response. These can be better seen in the computer software interface.
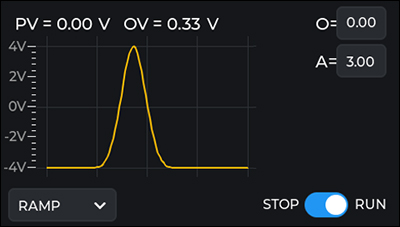
Recommended System Requirements | |
---|---|
Operating System | Windows® 10 (Recommended) or 11, 64 Bit Required |
Memory (RAM) | 4 GB Minimum, 8 GB Recommended |
Storage | 300 MB (Min) of Available Disk Space |
Screen Resolution | 1200 x 800 Pixels (Min) |
Interface | USB 2.0 |
Software
Version 1.0.0.0 (October 21, 2024)
Click the button below to visit the Digital Servo Controller software page.
Software for the DSC1 Compact Digital Servo Controller
The digital servo controller software is designed to both allow for control over basic functionality via a computer interface and provides an expanded set of analysis tools for using the controller. For example, the GUI includes a plot that can display the input voltage in frequency domain. Additionally, data can be exported as a .csv file.
This software allows for use of the device in the servo, peak, or ramp modes with control over all parameters and settings. The system response may be viewed as the input voltage, error signal, or both, either in the time domain or frequency domain representations. Please see the manual for more information.
The Servo tab allows a user to operate the device in servo mode with additional controls and displays beyond those provided by the embedded touchscreen user interface on the device itself. On this tab, either time or frequency domain representations of the process variable are available. The system response may be viewed as either the process variable, error signal, or both. The error signal is the difference between the process variable and the setpoint. Parameters including the P, I, and D coefficients can be adjusted from this interface. Changing the sign of the P, I, and D coefficients switches from locking on a positive slope to a negative slope and vice versa.
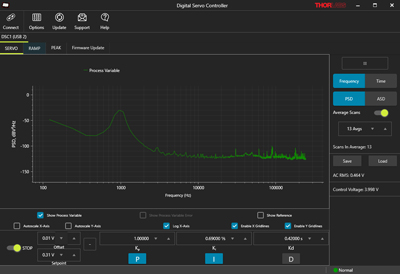
Click to Enlarge
The digital servo controller software interface in Servo mode can control all parameters of the lock as well as display the input voltage in either the time domain or frequency domain.
The Peak tab provides the same functionality as the peak mode on the embedded user interface, with additional visibility into the nature of the return signal from the system. Switching to this tab switches the connected device to the Peak mode of operation. Parameters including A, the amplitude of the sinusoidal modulation voltage, and K, the peak lock integral coefficient, can be changed. Changing the sign of K will determine if the controller locks onto a local peak or valley in the input voltage.
Similarly to Servo Lock mode, the demodulated input voltage may be viewed in either the time domain or frequency domain.
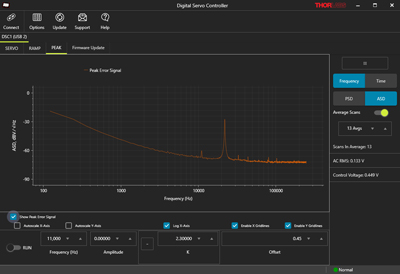
Click to Enlarge
The digital servo controller software interface in Peak mode can control all parameters of the lock as well as display the demodulated input voltage in the time domain or frequency domain.
The Ramp tab provides comparable functionality to the ramp tab on the embedded touchscreen display. Switching to this tab puts the connected device in ramp mode.
The input signal is plotted as a function of the ramp voltage allowing for the response of the system to be monitored in the same way that an oscilloscope would be used. As the x-axis is plotted in output volts, this can be used to determine where in the voltage range a lock should be configured.
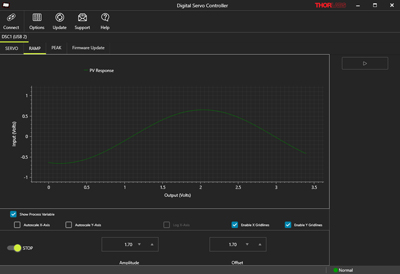
Click to Enlarge
The digital servo controller software interface in Ramp mode can control all parameters of the voltage sweep and display the response of the system as a function of voltage, alleviating the necessity for a separate oscilloscope.
Pin Diagrams for the DSC1 Digital Servo Controller
Signal Input and Output
SMB Female
This input accepts a ±4 V voltage maximum signal with a maximum current of 100 mA. It is internally terminated with a 1 MΩ impedance. To achieve lower input impedances, a BNC feed-through terminator can be added on.
Power Input
USB Type-C
USB Type-C to Type-A Power Input Cable Included
Computer Connection
USB Type Mini-B
USB Type Mini-B to Type-A Data Cable Included
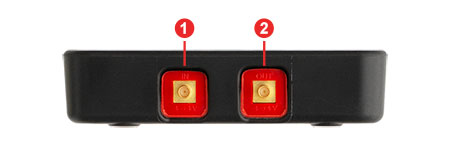
Click to Enlarge
DSC1 Compact Digital Servo Controller Right Panel
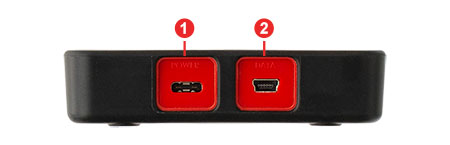
Click to Enlarge
DSC1 Compact Digital Servo Controller Left Panel
Left Panel | |
---|---|
Call Out | Description |
1 | Power Input (USB Type-C) |
2 | Computer Connection (USB Type Mini-B) |
Right Panel | |
---|---|
Call Out | Description |
1 | Signal Input, ±4 V (SMB Female) |
2 | Signal Output, ±4 V (SMB Female) |
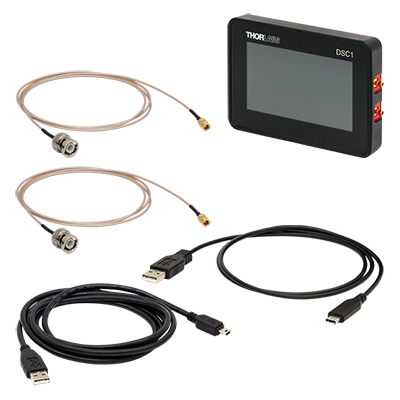
Click to Enlarge
Components Included with the DSC1 Compact Digital Servo Controller
Components Included with the DSC1 Compact Digital Servo Controller
Laser Locking
Below are three schematic examples of how a digital servo controller could be used to frequency lock a laser.
A laser can be frequency-stabilized by locking it to an atomic or molecular absorption line. In the setup depicted to the right, a ULN15TK narrow linewidth laser is passed through a vapor reference cell filled with acetylene. A balanced photodiode (PD) measures the transmission of the laser through the acetylene cell subtracted from the intensity of the input light, generating a signal proportional to the absorption by the narrow electronic transition of the acetylene. The output from the photodiode is fed into the digital servo controller (DSC) which generates a locking signal output that is fed into the laser modulation input, causing the laser frequency to be adjusted to maintain a fixed frequency relative to the absorption peak. The digital servo controller can be used in Peak Lock mode to lock the frequency of the laser to the peak of an absorption line or in Servo Lock mode to lock to an arbitrary height on one side of the absorption line, a side-of-fringe lock like used in Thorlabs' frequency locked laser. See the Frequency Lock tab on that page for more information on frequency locking to molecular absorption lines.
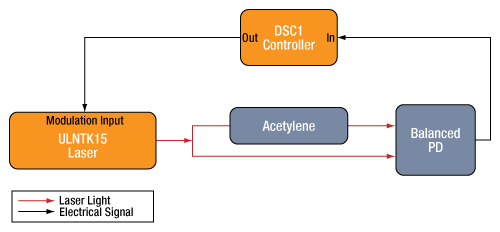
A schematic diagram for frequency locking a laser to a molecular absorption line using a photodiode (PD) and a DSC1 digital servo controller.
A Fabry-Perot optical cavity can be used to frequency stabilize and narrow the linewidth of a laser using a digital servo controller. In the setup depicted to the right, a single-frequency laser is sent into a Pound-Drever-Hall (PDH) system and on into a Fabry-Perot cavity. The Pound-Drever-Hall system collects light not retained in the cavity and creates an error signal that contains a steep zero-crossing in optical frequency at the peak of a cavity transmission fringe, allowing for robust locking. The PDH error signal is then fed into the digital servo controller (DSC) running in Servo Lock mode, which in turn manipulates the modulation input of the laser diode driver to lock the frequency of the light to the maximum of the laser cavity transmission.
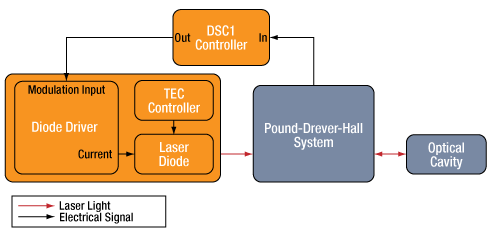
A schematic diagram for frequency locking a laser to an optical cavity using a Pound-Drever-Hall system and a DSC1 digital servo controller.
Transferring Stability Between Lasers Using a Fabry-Perot Cavity
A Fabry-Perot cavity (FP Cavity) can also be used as a transfer cavity lock to transfer the stability of one laser to another. In the setup depicted to the right, an atomically (or molecularly) referenced laser is fed into a Pound-Drever-Hall (PDH) system and on into a tunable Fabry-Perot cavity. The error signal from the PDH photodiode (PD) is fed into the first digital servo controller (DSC) which in turn manipulates the length of the Fabry-Perot cavity through the piezo driver (PZT Driver). This locks a cavity resonance, or fringe, to the frequency of the atomically stabilized laser. This stabilizes all the resonances of the cavity to the absorption feature used in the reference laser.
The secondary laser, which we are transferring atomically referenced stability to but can be a much different wavelength, is fed through the same Fabry-Perot cavity where a photodiode (PD) is used to measure its transmission through the PDH-stabilized cavity. The output from the photodiode is fed into the second digital servo controller (DSC) which manipulates the laser to maximize transmission through the cavity. In this example, the secondary laser frequency can be locked to the stabilized cavity using either a side-of-fringe lock or a peak lock. This locks the laser to the maximum transmission mode of the PDH-stabilized cavity, thereby transferring the frequency stability of the atomically referenced laser.
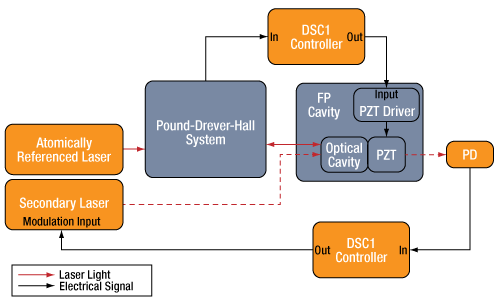
A schematic diagram for transferring stability between lasers using a Pound-Drever-Hall system, a Fabry-Perot cavity (FP Cavity) with piezo (PZT) tunability, a photodiode (PD), and two DSC1 digital servo controllers.
PIDの基礎
PID回路は制御ループフィードバックコントローラとしてよく用いられており、さまざまなサーボ回路として広く使われています。 PIDとは、それぞれ比例(Proportional)、積分(Integral)、微分(Derivative)の頭文字で、PID回路の3つの制御設定を表しています。 サーボ回路の役割は、システムを長時間所定値(目標値)に保持することです。 PID回路は、出力を目標値に保持するため、主に目標値と出力値の差をエラー信号として発生させることにより、システムをアクティブ制御しています。 3つの制御は、時間依存型エラー信号に関連しています; 端的に言うと、次のように考えることができます。 比例は出力値のエラー、積分は過去の累積エラー、微分はエラーの予測によっています。 各制御の結果は、その後回路の電流を調整する加重和にフィードされます(u(t))。 この出力は制御デバイスへ送られ、その値は回路へとフィードバックされ、回路の出力を目標値に到達させ保持するようアクティブ安定化の処理が行われます。 以下のブロック図は、PID回路の動作を簡略化したものです。 システム要求や要件によって、サーボ回路に1つもしくは複数の制御を使用することができます(例: P、I、PI、PD、PID)。
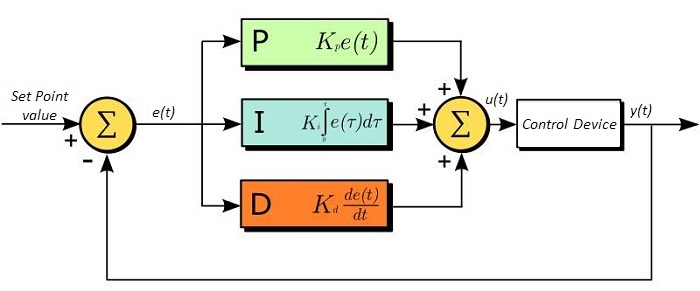
PID回路の適正な制御設定によって、最小限のオーバーシュート(目標値超過)とリンギング(目標値振動)で、素早い応答速度を実現できます。 ここで半導体レーザの温度安定化に用いられる温度サーボを例にとってみましょう。 PID回路は、最終的には熱電冷却素子(TEC)への電流を自動制御します(多くの場合FET回路上のゲート電圧の制御を通して行われます)。 この例では、電流は操作変数(MV)とします。 サーミスタは半導体レーザの温度モニタとして用いられ、サーミスタにかかる電圧を処理変数(PV)とします。 目標値(SP)の電圧は指定の温度に対応して設定します。 エラー信号e(t)は、SPとPVの差分を表します。 PIDコントローラはエラー信号を発生し、目標値に到達するようMVを変更させます。 例えばもし、e(t)の状態が半導体レーザの過熱を示せば、回路はTECを通してさらに電流を流すよう促します(比例制御)。 比例制御はe(t)に比例するので、半導体レーザを十分な速度で冷却できないかもしれません。 その場合、累積エラーから判断し、目標値へ到達させようと出力を調整し、回路はTECを介してさらに電流量を増加させます(積分制御)。 SPに到達すると(e(t)が0に近づくと)、回路はSPに達するのを見越してTECを通して電流を減少させます(微分制御)。
PID回路は適切な制御を保証するものではないことにご注意ください。 不適切なPID制御の設定は、回路を著しく振動させたり、制御の不安定を引き起こす可能性があります。 正しい動作は、PIDの適正な調整によって得られます。
PID理論
PID制御回路u(t)の出力を得る方程式は以下となります。

Kp= 比例利得
Ki = 積分利得
Kd =微分利得
e(t)=SP-PV(t)
ここから制御ユニットは数学的定義によって定義づけることができ、個々の制御についてもう少し詳しく考察することができます。 比例制御は、エラー信号に比例します。これは、回路が発生させたエラー信号に対する直接的な応答です。
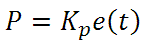
より大きな比例利得は、より大きな変化をエラーへの応答にもたらし、コントローラがシステムの変化に応答できる速度に影響を与えます。 比例利得の値が高いと回路の応答を素早く行えますが、あまりに高い場合は、SP値に対して振動を引き起こしてしまいます。 値が低すぎる場合は、回路はシステム変更への応答性が悪くなります。
積分制御は、比例利得よりさらに1段階ステップが進み、エラー信号の大きさだけでなく、エラーの期間にも比例しています。
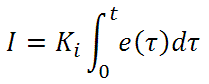
積分制御は、比例制御のみによる定常誤差を除去するとともに、回路の応答速度向上に非常に高い効果をもたらします。 積分制御は、未修正の過去のエラーを合計し、エラーにKiを乗算することで、積分応答を出します。 従ってわずかな継続エラーに対しても、大規模な集積積分応答を実現することが可能です。 しかしながら、積分制御の高速応答に起因して、高い利得値による目標値の著しい超過が生じ、振動と不安定性を引き起こします。 低すぎる場合、回路のシステム変更への応答速度が著しく低下します。
微分制御は、比例制御および積分制御から予測される目標値超過とリンギングを低減させます。 回路が時間の経過とともにどう変化しているか(エラー信号の微分から判断)素早く決定し、Kdを乗算することで微分応答を出します。
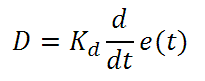
比例や積分制御と異なり、微分制御は回路の応答を減速させます。 そのため、積分制御や比例制御によって引き起こされた振動を抑制したり、超過を部分的に補うことができます。 高い利得値は回路の応答性にかなりの減速を生じさせ、ノイズや高周波振動が発生しやすくなります(回路が迅速に応答するには低速すぎるため)。 低すぎると、回路はSP値を超過する傾向にあります。しかしながら、SP値を著しく超過するケースは避けなければならず、そのためより高い微分利得(より低い比例利得とともに)が用いられます。 下記の図は、個々のパラメータの利得の増加による影響を示しています。
Parameter Increased | Rise Time | Overshoot | Settling Time | Steady-State Error | Stability |
---|---|---|---|---|---|
Kp | Decrease | Increase | Small Change | Decrease | Degrade |
Ki | Decrease | Increase | Increase | Decrease Significantly | Degrade |
Kd | Minor Decrease | Minor Decrease | Minor Decrease | No Effect | Improve (for small Kd) |
チューニング
通常、適切なサーボ制御を得るために、P、I、Dの利得値は個々で調整する必要があります。 どのシステムに対してもどの値にするべき、といった決まった一連のルールがあるわけではありませんが、基本手順に沿ったチューニングは各々のシステムや環境に合わせるのに役立ちます。 概して、PID回路はSP値の超過をわずかに起こし、その後SP値に到達させるため素早く減衰するようにします。
手動による利得設定のチューニングは、PID制御設定において最もシンプルな方法です。 しかしながらこの手順はアクティブで行われ(PIDコントローラがオンとなり、システムに正しく接続されている)、完全に設定するには多少の経験を要します。 PIDコントローラを手動で調整するには、まず始めに積分および微分利得を0に設定します。 出力に振動が現れるまで、比例利得を上げてください。 比例利得はこの値の約半分の値に設定します。 比例ゲイン利得設定後は、任意のオフセットがシステムに合わせた適切なタイムスケールに修正されるまで積分利得を上げてください。 上げすぎた場合は、SP値の著しい超過と回路の不安定性が引き起こされます。 積分利得が設定されたら、次に微分利得を上げてください。 微分利得はオーバーシュートを軽減し、システムを迅速にSP値へ収束させます。 微分利得を上げすぎると、大幅な超過が生じます(回路の応答が低速すぎるため)。利得設定を試行することにより、システムが変化へ素早く応答し、SP値の振動を効率よく減衰させるといった、PID回路の性能を最大限にすることができます。
Control Type | Kp | Ki | Kd |
---|---|---|---|
P | 0.50 Ku | - | - |
PI | 0.45 Ku | 1.2 Kp/Pu | - |
PID | 0.60 Ku | 2 Kp/Pu | KpPu/8 |
手動によるチューニングは非常に効果的なPID回路の設定方法ですが、ある程度の経験とPID回路および応答についての理解を必要とします。 PIDチューニングのためのZiegler-Nicholsメソッドは、もう少し体系的な手引きとなっています。 再び、積分利得と微分利得をゼロ値にセットしてください。 比例利得を回路が振動するまで上げます。 この利得をレベルKuと呼びます。 振動はPuの期間です。 個々の制御回路の各利得は右の表に示しています。
Posted Comments: | |
Diogo Almeda
 (posted 2024-10-30 14:03:04.39) Hello,
I work with coherent ultrafast spectroscopy and my experiment currently uses 3 servo loops for optical paths’ length stabilization and control. I noticed Thorlbas recently started selling a compact PID servo solution (DSC1) that could be a very good alternative to the hard-to-service loops I built myself. The complication involving my setup is that I need to modify the optical paths and take data at a very specific order and that would require an API, SDK or external control from you so I could, at least, turn on/off the DSC1 lock on the fly.
As far as I could check in the manual, that is not currently possible. So I would like to ask if there is any short-term plans for LabView/Python support release.
Best,
Diogo |

- High-Speed PID Control with up to 100 kHz Bandwidth
- Ideal for Frequency Stabilizing Lasers
Thorlabs' DSC1 Compact Digital Servo Controller locks or stabilizes an optical system to an input voltage signal with real-time voltage feedback determined by a user-selected control algorithm. The controller has three modes that offer complementary functionality: Servo Lock mode, Peak Lock mode, and Ramp mode. The Servo Lock mode locks a system to maintain a constant specified value of the input voltage. The Peak Lock mode locks a system to a local peak or valley in the input voltage. The Ramp mode linearly sweeps the output voltage over a defined range for observation of the system's response.
The DSC1 controller includes two PAA248 SMB to BNC 48” (1.22 mm) long coaxial cables, one USB-AB-72 USB 2.0 Type-A to Mini-B 72" (1.83 m) long data cable, and one USB Type-A to USB Type-C 1 m (39") long power cable. A power supply is sold separately; the DS5 Regulated Power Supply or CPS1 Battery Pack, sold below, is recommended for powering the DSC1 controller.

- DS5 Power Supply Provides Power From a Wall Outlet
- CPS1 Battery Pack Allows for Lower Noise with an 8 Hour or Greater Run Time
The DS5 Regulated Power Supply or CPS1 Battery Pack is recommended for powering the DSC1 controller. In applications where runtimes of greater than 8 hours are required, the DS5 regulated power supply is the preferred option. When optimal noise performance is desired, the CPS1 battery pack provides cleaner power for a lower noise floor.
DS5 Power Supply
The DS5 power supply is a 5 V, 2 A regulated power supply with a USB Type-A female port that can be used with any USB-compatible device that accepts a 5 VDC output. A region-specific adapter plug is shipped with the DS5 power supply unit based on your location.
CPS1 Battery Pack
In applications where lower noise is required, the CPS1 battery pack offers improved performance in exchange for limited run time. With the CPS1 battery pack fully charged and in good health, the DSC1 controller can operate for 8 hours or more without recharging. The CPS1 battery pack outputs 5 VDC at up to 2 A through a USB Type-A female port and offers 10 000 mAh of capacity. The pack includes a USB Type-A to Micro-B cable for charging. To activate the battery, simply push the power button once. The pack may be charged using standard 5 V USB chargers for portable devices or using a computer USB port. While the battery pack is charging, it can still supply power to an attached device.